Polyvagal Theory A Primer
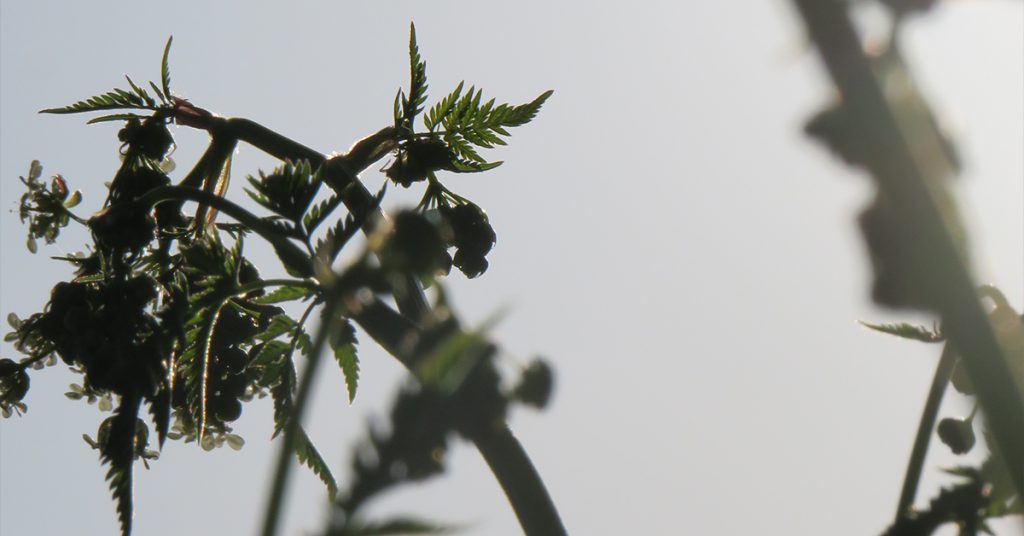
Abstract This chapter provides an overview of the Polyvagal Theory. The chapter is organized to facilitate an understanding of the constructs embedded in the theory that are relevant for clinical application. The chapter is organized with headings identifying important constructs within the theory, many of which are used by the authors of the chapters that follow.
Although Polyvagal Theory is the focus of this volume, the contributing authors were requested not to restate the details of the theory within their chapters. Instead, this chapter provides an overview of the theory. Although this chapter is dense and scientific, we hoped that by providing information on the theory in beginning of the book, we would allow the authors to be less encumbered by the science and to write in a more personal voice that would convey how Polyvagal Theory influenced their work. To facilitate the generalizability of Polyvagal Theory to clinical application, the chapter is organized with headings identifying important constructs within the theory. Polyvagal Theory describes an autonomic nervous system that is influenced by the central nervous system and responds to signals from both the environment and bodily organs. The theory emphasizes that the human autonomic nervous system has a predictable pattern of reactivity, which is dependent on neuroanatomical and neurophysiological changes that occurred during evolution. Specifically, the theory focuses on the phylogenetic changes in the neural regulation of bodily organs during the evolutionary transition from ancient extinct reptiles to the earliest mammals.
Evolution of the Vertebrate Autonomic Nervous System: As mammals evolved, their behaviors differentiated them from their primitive reptilian ancestors. Unlike the solitary behaviors and lack of nurturance of their vertebrate ancestors, mammals expressed a broad range of social behaviors, including caring for offspring and cooperation. These behaviors supported the survival of mammals. However, in order for these behaviors to occur, the mammalian nervous system had to selectively down regulate defensive reactions. This convergence was dependent on the coevolution of modifications in the neural regulation of the autonomic nervous system and the sociality that defines mammalian behavior.
To understand Polyvagal Theory, it is first necessary to understand three contingent points: first, the relationship between autonomic state and defensive behaviors; second, the changes that occurred during vertebrate evolution in the neural regulation of the autonomic nervous system; and third, the physiological state, which enables bodily responses and feelings of safety, optimizes social behavior and concurrently optimizes health, growth, and restoration.
In most vertebrates, the two primary defense systems are fight- or- flight and immobilization. Fight- or- flight behaviors enable the organism to flee or defend when threatened. These behaviors require the rapid accessibility of resources to mobilize through the activation of the metabolically costly sympathetic nervous system. Immobilization is a more ancient defense system, which is shared with virtually all vertebrates. In contrast to the metabolically costly mobilization strategy, immobilization is an adaptive attempt to reduce metabolic demands (e.g., reduced options for food and oxygen) and to appear inanimate (e.g., death feigning). Juxtaposed with the rapid activation of the sympathetic nervous system required to promote fight- or- flight behaviors, immobilization defense behaviors required a massive shutting down of autonomic function via a vagal pathway within the parasympathetic nervous system.
Over time, a second vagal pathway evolved that had the capacity to down regulate both forms of defense. This second vagal pathway is observed in mammals and not reptiles. In addition, the anatomical structures regulating this component of the vagus interacted in the brain stem with structures regulating the striated muscles of the face and head to provide an integrated social engagement system. This emergent social engagement system provided the mechanism for co- regulation of physiological state, as mammals conveyed cues of safety and danger— via vocalizations, head gestures, and facial expressions— to conspecifics. The social engagement system enabled mammals to co- opt some of the features of the vertebrate defense systems to promote social interactions such as play and intimacy. These changes in the autonomic nervous system provided mammals with neural mechanisms to promote the biobehavioral states necessary for caring for offspring, reproducing, and cooperative behavior. In contrast, the adverse behavioral and psychological effects of trauma appear to target a disruption of the social engagement system, its management of defense reactions, and its contribution to co- regulation and cooperative behaviors, including intimacy and play.
FIGURE4.1
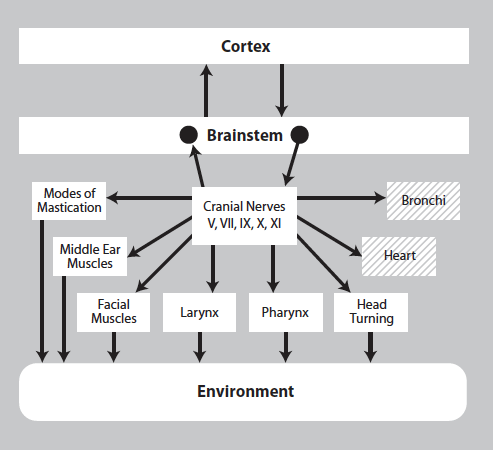
FIGURE 4.1 – The social engagement system consists of a somatomotor component (solid blocks) and a visceromotor component (dashed blocks). The somatomotor component involves special visceral efferent pathways that regulate the striated muscles of the face and head, while the visceromotor component involves the myelinated vagus that regulates the heart and bronchi.
Origin of Polyvagal Theory: The Vagal Paradox
Polyvagal Theory emerged from research studying heart rate patterns in human fetuses and newborns. In obstetrics and neonatology, the massive slowing of heart rate known as bradycardia is a clinical index of risk and assumed to be mediated by the vagus. During bradycardia, heart rate is so slow that it no longer provides sufficient oxygenated blood to the brain. This type of vagal influence on the fetal and neonatal heart could potentially be lethal. However, with the same clinical populations, a different index of vagal function was assumed to be a measure of resilience. This measure was beat- to- beat heart rate variability and was the focus of my research for several decades. Animal research demonstrated that both signals could be disrupted by severing the vagal pathways to the heart or via pharmacological blockade (i.e., atropine), interfering with the inhibitory action of the vagus on the sinoatrial node (for review see Porges, 1995). These observations posed the paradox of how cardiac vagal tone could be both a positive indicator of health when monitored with heart rate variability and a negative indicator of health when it manifests as bradycardia.
The resolution to the paradox came from understanding how the neural regulation of the autonomic nervous system changed during evolution, especially through the transition from primitive extinct reptiles to mammals. During this transition, mammals evolved a second vagal motor pathway. This uniquely mammalian pathway is myelinated and conveys a respiratory rhythm to the heart’s pacemaker, resulting in a rhythmic oscillation in heart rate at the frequency of spontaneous breathing known as respiratory sinus arrhythmia. Myelin is a fatty substance that surrounds the fiber. Myelin provides electrical insulation for the fiber, which enables the signal to be transmitted with greater specificity and speed. This branch of the vagus originates in an area of brain stem known as the nucleus ambiguus, travels primarily to organs above
the diaphragm, and interacts within the brain stem with structures regulating the striated muscles of the face and head. The other vagal motor pathway does not have a respiratory rhythm, is observed in virtually all vertebrates, is unmyelinated, travels primarily to organs below the diaphragm, and originates in an area of the brain stem known as the dorsal nucleus of the vagus.
Phylogenetic Shifts in Vertebrate Autonomic Nervous Systems
By tracking the evolutionary changes in the vertebrate autonomic nervous system, I identified a phylogenetic pattern consisting of three evolutionary stages. During the first stage, vertebrates relied on an unmyelinated vagus with motor pathways originating in an area of the brain stem resembling the dorsal vagal complex. During the second stage, an excitatory spinal sympathetic nervous system developed, which complemented the down regulation functions of the ancient vagal pathway. During the third stage, defined by the emergence of mammals, an additional vagal pathway evolved, during which cells of origin of the vagus migrated from the dorsal nucleus of the vagus to the nucleus ambiguus; many of the vagal motor fibers originating in the nucleus ambiguus became myelinated and integrated in the function of the brain stem regulation of a family of motor pathways (i.e., special visceral efferent pathways) that control the striated muscles of the face and head (see Figure 4.1).
In mammals, the unmyelinated vagal pathways originating in the dorsal nucleus of the vagus primarily regulate the organs below the diaphragm, though some of these unmyelinated vagal fibers terminate on the heart’s pacemaker (sinoatrial node). Polyvagal Theory hypothesizes that these unmyelinated vagal fibers primarily remain dormant until life threat, and they are probably potentiated during hypoxia and states in which the influence of the myelinated vagal input to the heart is depressed. This sequence is observable in human fetal heart rate, in which bradycardia is more likely to occur when the tonic influence of the myelinated vagal pathways, manifest in respiratory sinus arrhythmia, is low (Reed, Ohel, David, & Porges, 1999). This also may be the mechanism mediating trauma- elicited dissociation, defecation, and syncope (i.e., fainting).
In ancient vertebrates, an unmyelinated vagal pathway emerging from the brain stem was a critical component of the neural regulation of the entire viscera. This bidirectional system reduced metabolic output when resources were low, such as during times of reduced oxygen. The nervous systems of primitive vertebrates did not need much oxygen to survive and could lower heart rate and metabolic demands when oxygen levels dropped. Thus, this circuit
provided a conservation system that in mammals was adapted as a primitive defense system manifested as death feigning and trauma- driven responses of syncope and dissociation. Since this defense system could be lethal in oxygen- demanding mammals, fortunately it functions as the last option for survival. The phylogenetically older unmyelinated vagal motor pathways are shared with most vertebrates and, in mammals, when not recruited as a defense system, function to support health, growth, and restoration via neural regulation of subdiaphragmatic organs (i.e., internal organs below the diaphragm).
The “newer” myelinated ventral vagal motor pathways regulate the supradiaphragmatic organs (e.g., heart and lungs) and are integrated in the brain stem with structures that regulate the striated muscles of the face and head via special visceral efferent pathways resulting in a functional social engagement system. This newer vagal circuit slows heart rate and supports the states of calmness required for social interactions. The ventral vagal circuit coupled with other autonomic circuits supports social play (i.e., ventral vagal coupled with sympathetic activation) and safe intimacy (i.e., ventral vagal coupled with dorsal vagal circuit). Thus, the mammalian vagus has properties that promote states that contain the range of responding of all components of the autonomic nervous system and functionally restrains the system from moving into states of defense.
The Emergence of the Social Engagement System
The integration of the myelinated cardiac vagal pathways with the neural regulation of the face and head gave rise to the mammalian social engagement system. As illustrated in Figure 4.1, the outputs of the social engagement system consist of motor pathways regulating striated muscles of the face and head (i.e., somatomotor) and smooth and cardiac muscles of the heart and bronchi (i.e., visceromotor). The somatomotor component involves special visceral efferent pathways that regulate the striated muscles of the face and head. The visceromotor component involves the myelinated supradiaphragmatic vagal pathway that regulates the heart and bronchi. Functionally, the social engagement system emerges from a face- heart connection that coordinates the heart with the muscles of the face and head. The initial function of the system is to coordinate sucking, swallowing, breathing, and vocalizing. Atypical coordination of this system early in life is an indicator of subsequent difficulties in social behavior and emotional regulation.
When fully developed, two important biobehavioral features of this system are expressed. First, bodily state is regulated in an efficient manner to promote growth and restoration (e.g., visceral homeostasis). Functionally, this is accom
plished through an increase in the influence of myelinated vagal motor pathways on the cardiac pacemaker to slow heart rate, inhibit the fight- or- flight mechanisms of the sympathetic nervous system, dampen the stress response system of the hypothalamic– pituitary– adrenal (HPA) axis (responsible for cortisol release), and reduce inflammation by modulating immune reactions (e.g., cytokines; for review see Porges, 2007). Second, the phylogenetically mammalian face– heart connection functions to convey physiological state
FIGURE4.1
The social engagement system consists of a somatomotor component (solid blocks) and a visceromotor component (dashed blocks). The somatomotor component involves special visceral efferent pathways that regulate the striated muscles of the face and head, while the visceromotor component involves the myelinated vagus that regulates the heart and bronchi.
via facial expression and prosody (intonation of voice), as well as regulate the middle- ear muscles to optimize species- specific listening within the frequency band used for social communication (Kolacz, Lewis, & Porges, in press; Porges, 2007, 2009, 2011; Porges & Lewis, 2010).
The brain stem source nuclei of the social engagement system are influenced by higher brain structures (i.e., top- down influences) and by sensory pathways from visceral organs (i.e., bottom- up influences). Direct pathways from cortex to brain stem (i.e., corticobulbar) reflect the influence of frontal areas of the cortex (i.e., upper motor neurons) on the medullary source nuclei of this system. Bottom- up influences occur via feedback through the sensory pathways of the vagus (e.g., tractus solitarius), conveying information from visceral organs to medullary areas (e.g., nucleus of the solitary tract) and influencing both the source nuclei of this system and the forebrain areas, via the insula, that are assumed to be involved in several psychiatric disorders, including depression and anxiety (Craig, 2005; Thayer & Lane, 2007, 2009). In addition, the anatomical structures involved in the social engagement system have neurophysiological interactions with the HPA axis, the social neuropeptides (e.g., oxytocin and vasopressin), and the immune system (Carter, 1998; Porges, 2001).
Sensory pathways from the target organs of the social engagement system, including the muscles of the face and head, also provide potent input to the source nuclei regulating both the visceral and somatic components of the social engagement system. The source nucleus of the facial nerve forms the border of nucleus ambiguus, and sensory pathways from both the facial and trigeminal nerves provide a primary sensory input to nucleus ambiguus (see Porges, 1995, 2007). Thus, the ventral vagal complex, consisting of nucleus ambiguus and the nuclei of the trigeminal and facial nerves, is functionally related to the expression and experience of affective states and emotions. Activation of the somatomotor component (e.g., listening, ingestion, vocalizations, facial expressions) could trigger visceral changes that would support social engagement, while modulation of visceral state, depending on whether there is an increase or decrease in the influence of the myelinated vagal motor fibers on the sinoatrial node (i.e., increasing or decreasing the influence of the vagal brake), would either promote or impede social engagement behaviors (Porges, 1995, 2007). For example, stimulation of visceral states that promote mobilization (i.e., fight- or- flight behaviors) would impede the ability to express social engagement behaviors.
The face– heart connection enabled mammals to detect whether a conspecific was in a calm physiological state and safe to approach, or in a highly mobilized and reactive physiological state during which engagement would be dangerous. The face– heart connection concurrently enables an individual to signal “safety” through patterns of facial expression and vocal intonation, and potentially calm an agitated conspecific to form a social relationship. When the newer mammalian vagus is optimally functioning in social interactions, emotions are well regulated, vocal prosody is rich, and the autonomic state supports calm, spontaneous social engagement behaviors. The face– heart system is bidirectional, with the newer myelinated vagal circuit influencing social interactions and positive social interactions influencing vagal function to optimize health, dampen stress- related physiological states, and support growth and restoration. Social communication and the ability to co- regulate interactions, via reciprocal social engagement systems, lead to a sense of connectedness and are important defining features of the human experience.
Vagal Brake
The vagal brake reflects the tonic inhibitory influence of the myelinated vagal pathways on the heart, which slows the intrinsic rate of the heart’s pacemaker. The intrinsic heart rate of young, healthy adults is about 90 beats per minute. However, baseline heart rate is noticeably slower due to the influence of the vagus, which functions as a “vagal brake.” When the vagus decreases its influence on the heart (i.e., the vagal brake releases), heart rate spontaneously increases. This is not due to an increase in sympathetic excitation; rather, the release of the vagal brake allows the rate intrinsic in the pacemaker to be expressed. The vagal brake represents the actions of engaging and disengaging the vagal influences to the heart’s pacemaker. In addition, the release of the vagal brake on the heart also enables tonic underlying sympathetic excitation to exert more influence on the autonomic nervous system. Polyvagal Theory specifically assumes that the vagal brake is mediated solely through the myelinated ventral vagus and can be quantified by the amplitude of respiratory sinus arrhythmia. The theory acknowledges other neural (e.g., dorsal vagal pathways) and neurochemical influences that can slow heart rate (e.g., clinical bradycardia), which are not included within the construct of the vagal brake.
Dissolution
The human nervous system, similar to that of other mammals, evolved not solely to survive in safe environments, but also to promote survival in dangerous and life- threatening contexts. To accomplish this adaptive flexibility, the mammalian autonomic nervous system, in addition to the myelinated vagal pathway that is integrated into the social engagement system, retained two more primitive neural circuits to regulate defensive strategies (i.e., fight- or- flight and death- feigning behaviors). It is important to note that social behavior, social communication, and visceral homeostasis are incompatible with the neurophysiological states that support defense. Polyvagal response strategies to challenge are phylogenetically ordered, with newest components of the autonomic nervous system responding first. This model of autonomic reactivity is consistent with John Hughlings Jackson’s construct of dissolution, in which he proposed that “the higher nervous arrangements inhibit (or control) the lower, and thus, when the higher are suddenly rendered functionless, the lower rise in activity” (1882, p. 412). In this hierarchy of adaptive responses, the newest social engagement circuit is used first; if that circuit fails to provide safety, the older circuits are recruited sequentially.
Neuroception
Polyvagal Theory proposes that the neural evaluation of risk does not require conscious awareness and functions through neural circuits that are shared with our phylogenetic vertebrate ancestors. Thus, the term neuroception was introduced to emphasize a neural process, distinct from perception, capable of distinguishing environmental and visceral features that are safe, dangerous, or life- threatening (Porges, 2003, 2004). In safe environments, autonomic state is adaptively regulated to dampen sympathetic activation and to protect the oxygen- dependent central nervous system, especially the cortex, from the metabolically conservative reactions of the dorsal vagal complex (e.g., fainting).
Neuroception is proposed as a reflexive mechanism capable of instantaneously shifting physiological state. Feature detectors, located in areas of or near the temporal cortex, which are sensitive to the intentionality of biological movements including voices, faces, gestures, and hand movements, might be involved in the process of neuroception. Embedded in the construct of neuroception is the capacity of the nervous system to react to the “intention” of these movements. Neuroception functionally decodes and interprets the assumed goal of movements and sounds of inanimate and living objects. Thus, the neuroception of familiar individuals and individuals with appropriately prosodic voices and warm, expressive faces frequently translates into a positive social interaction, promoting a sense of safety. Although we are often unaware of the stimuli that trigger different neuroceptive responses, we are generally aware of our body’s reactions.
Autonomic State as an Intervening Variable
Polyvagal Theory proposes that physiological state is a fundamental part, and not a correlate, of emotion or mood. According to the theory, autonomic state functions as an intervening variable biasing our detection and evaluation of environmental cues. Depending on physiological state, the same cues will be reflexively evaluated as neutral, positive, or threatening. Functionally, a change in state will shift access to different structures in the brain and support either social communication or the defensive behaviors of fight- or- flight or shutdown. Contemporary research on the impact of vagal nerve stimulation on cognitive function and emotion regulation supports this model (Groves & Brown, 2005). The theory emphasizes a bidirectional link between brain and viscera, which would explain how thoughts change physiology, and physiological state influences thoughts. As individuals change their facial expressions, the intonation of their voices, the pattern in which they are breathing, and their posture, they are also changing their physiology through circuits involving myelinated vagal pathways to the heart.
The Role of Sensations From Bodily Organs in the Regulation of Autonomic State
The prevalent focus of research investigating the neural regulation of the heart has focused on motor pathways emerging from brain stem nuclei (i.e., vagal pathways) and the sympathetic nervous system. Limited research has been conducted on the influence of sensory feedback from bodily organs (i.e., visceral afferents) in the neural regulation of the autonomic nervous system, and how these influences are manifested in the heart and other visceral organs. This is, in part, due to a top- down bias in medical education that limits the conceptualization of the neural regulation of the heart and other bodily organs by emphasizing the role of motor fibers and minimizing the role of sensory fibers. However, this bias is rapidly changing due to research on the applications of vagal nerve stimulation, a bottom- up model that focuses on the vagus as a sensory nerve (approximately 80% of the vagal fibers are sensory). Interestingly, the side effects of vagal nerve stimulation are frequently due to the influence of vagal nerve stimulation on motor pathways. These side effects are primarily noted on features of the social engagement system, including changes in voice and difficulties swallowing (Ben- Menachem, 2001). However, in some cases, the stimulation has been manifested in subdiaphragmatic organs, resulting in diarrhea (Sanossian & Haut, 2002). As vagal nerve stimulation becomes more commonly applied to medical disorders, there is an
emerging awareness of the influence of the sensory pathways of the vagus on neurophysiological function (e.g., epilepsy), emotional state (e.g., depression), and cognition (e.g., learning and attention; Howland, 2014).
According to Polyvagal Theory, the social engagement system is regulated by complex neural circuits, involving both sensory pathways from visceral organs (i.e., bottom- up) and higher brain structures (i.e., top- down) that influence the brain stem source nuclei controlling both the myelinated vagus and the striated muscles of the face and head. As the surveillance role of sensory pathways providing feedback from bodily organs to the brain stem is incorporated into an understanding of the autonomic nervous system, clinicians and researchers will begin to recognize manifestations in the vagal control of the heart in patients with a variety of disorders of peripheral organs. With that understanding, rather than interpreting the atypical neural regulation of the heart as a cardiovascular disease, comorbidities may be explained as manifestations of “system” dysfunction consistent with the prescient views of Walter Hess (1949/2014).
Several chronic diseases manifested in specific subdiaphragmatic organs (e.g., kidney, pancreas, liver, gut, genitals, etc.) have identifiable features that have led to treatments that target organs (e.g., medication, surgery). However, other disorders that have an impact on quality of life, such as irritable bowel syndrome and fibromyalgia, are defined by nonspecific symptoms. The literature links these nonspecific chronic disorders with atypical vagal regulation of the heart, reflected in diminished heart rate variability (Mazurak, Seredyuk, Sauer, Teufel, & Enck, 2012; Staud, 2008). Consistent with these findings, heart rate variability has been proposed as a biomarker for these disorders.
Polyvagal Theory proposes an alternative interpretation of this covariation. Consistent with the integrated model of the autonomic nervous system described in the theory, atypical heart rate variability is not interpreted as a biomarker of any specific disease. Rather, depressed heart rate variability is proposed as a neurophysiological marker of a diffuse retuning of the autonomic nervous system, indicating a withdrawal of the ventral vagal circuit following an adaptive complex autonomic reaction to threat. Compatible with this interpretation, there are strong links between the prevalence of a history of abuse, especially sexual abuse in women, and the manifestations of nonspecific clinical disorders such as irritable bowel syndrome and fibromyalgia. In addition, emotional stress intensifies symptoms and hinders positive treatment outcomes, and trauma may trigger or aggravate symptoms (Clauw, 2014; Whitehead et al., 2007). The initial adaptive neural response to threat, via sensory feedback from the visceral organs to the brain stem, may result in a chronic reorganization of the autonomic regulation observed in vagal regulation of the heart (i.e., depressed heart rate variability) in conjunction with altered subdiaphragmatic organ function and pain signaling.
A New Perspective of the Autonomic Nervous System
Polyvagal Theory uses an inclusive definition of the autonomic nervous system that includes sensory pathways and emphasizes the brain stem areas regulating autonomic function. The theory links the brain stem regulation of the ventral vagus to the regulation of the striated muscles of the face and head to produce an integrated social engagement system (see Figure 4.1 on the ventral vagal complex and social engagement system).
In contrast to the traditional model that focuses on tonic motor influences on visceral organs, Polyvagal Theory emphasizes autonomic reactivity. Polyvagal Theory accepts the traditional model of interpreting tonic autonomic influences on several visceral organs as the sum of a paired antagonism between vagal and sympathetic pathways. However, Polyvagal Theory proposes a phylogenetically ordered hierarchy in which autonomic subsystems react to challenges in the reverse of their evolutionary history, consistent with the principle of dissolution.
The theory postulates that when the ventral vagus and the associated social engagement system are optimally functioning, the autonomic nervous system supports health, growth, and restoration. During this ventral vagal state, there is an optimal “autonomic balance” between the sympathetic nervous system and the dorsal vagal pathways to subdiaphragmatic organs. When the function of the ventral vagus is dampened or withdrawn, the autonomic nervous system is optimized to support defense, and not health. According to Polyvagal Theory, these defense reactions may be manifested as fight- or- flight or shutdown. As fight- or- flight defense, there is an increase in sympathetic activity to promote mobilization strategies while inhibiting digestion (and other dorsal vagal functions). In contrast, when manifested as shutdown, sympathetic activation is depressed, while there is a surge of the dorsal vagal influences that would promote fainting, defecation, and an inhibition of motor behavior often seen in mammals feigning death.
Cues of Safety Are the Treatment
Polyvagal Theory proposes that cues of safety are an efficient and profound antidote for trauma. The theory emphasizes that safety is defined by feeling safe and not simply by the removal of threat. Feeling safe is dependent on three conditions: 1) the autonomic nervous system cannot be in a state that supports defense; 2) the social engagement system needs to be activated to down regulate sympathetic activation and functionally contain the sympathetic nervous system and the dorsal vagal circuit within an optimal range (homeostasis) that would support health, growth, and restoration; and 3) cues of safety (e.g., prosodic vocalizations, positive facial expressions and gestures) need to be available and detected via neuroception. In everyday situations, the cues of safety may initiate the sequence by triggering the social engagement system via the process of neuroception, which will contain autonomic state within a homeostatic range and restrict the autonomic nervous system from reacting in defense. This constrained range of autonomic state has been referred to as the window of tolerance (see Ogden, Minton, & Pain, 2006; Siegel, 1999) and can be expanded through neural exercises embedded in therapy.
Neural Exercise as Intervention
Polyvagal Theory focuses on specific neural exercises that provide opportunities to optimize the regulation of physiological state. According to the theory, neural exercises consisting of transitory disruptions and repairs of physiological state through social interactions employing cues of safety would promote greater resilience. Play, such as peek- a- boo, is an example of a neural exercise that parents frequently employ with their children. Play provides an example of a therapeutic model in which autonomic state is disrupted and then stabilized through the recruitment of the social engagement system. This model can be generalized to the clinical setting, in which the client experiences disrupted changes in autonomic state, which are stabilized through the support of the therapist. Functionally, therapy becomes a platform to exercise the capacity to shift state by recruiting features of the social engagement system to keep the autonomic nervous system out of prolonged states of defense. This process is initialized through co- regulation between the client and the therapist. Subsequently, when the client experiences reliable co- regulation, the potency of transitory shifts in state as triggers of defense is reduced and self- regulation spontaneously emerges.
Through the metaphor of play, the social engagement system is coupled with the sympathetic nervous system. This coupling enables bodily cues of mobilization to be contained within a social setting and not to erupt into aggression. However, these eruptions or tantrums frequently occur in children and adults with behavioral problems and psychiatric disorders. Research documents a consistency of a down regulated social engagement system (e.g., lack of prosody, blunted facial expression, auditory hypersensitivities, poor eye gaze) in individuals with state regulation disorders (see Porges, 2011).
Polyvagal Theory emphasizes that the vulnerability to these disruptions is due to a physiological state shift characterized by sympathetic activation without the resource of efficient self- soothing or calming through the social engagement system.
The metaphor of play is also useful in deconstructing intimacy. Intimacy is a state- dependent behavior. Intimacy involves coupling the social engagement system with the dorsal vagal circuit to enable immobilization without shutdown. Intimacy requires a state in which touch and proximity do not trigger defense. For mammals, immobilization is a vulnerable state. For intimacy to occur, neuroception has to interpret proximity and contact as safe and shift the body into a state that is welcoming. This coupling of two bodies initially occurs through cues of safety, such as prosodic vocalizations and gentle contact. Intimacy is often associated with a form of play, foreplay. However, similar to the positive attributes of play, which functions as a neural exercise optimizing the ability of the social engagement system to regulate the sympathetic nervous system, foreplay and truly safe experiences of intimacy provide a neural exercise optimizing the ability of the social engagement system to regulate the dorsal vagal pathway. This form of neural exercise may have long- term beneficial effects on the regulation of bodily organs by supporting homeostasis. Moreover, safe foreplay and intimacy may also be a preparatory neural exercise for women that, by enabling immobilization without fear, would optimize the reproductive behaviors and processes, including facilitating childbirth.
Listening as a Neural Exercise
Polyvagal Theory emphasizes how listening is a portal to the social engagement system. Based on Polyvagal Theory, the Listening Project Protocol is a listening intervention designed to reduce auditory hypersensitivities, improve auditory processing, calm physiological state, and support spontaneous social engagement. The intervention is currently known as the Safe and Sound Protocol and is available to professionals only through Integrated Listening Systems (http://integratedlistening.com/ssp- safe- sound- protocol/).
The Safe and Sound Protocol is based on an “exercise” model that uses computer- altered acoustic stimulation to modulate the frequency band passed to the participant. The protocol was theoretically designed to reduce auditory hypersensitivities by recruiting the antimasking functions of the middle- ear muscles to optimize the transfer function of the middle ear for the processing of human speech. Modulation of the acoustic energy within the frequencies of human voice, similar to exaggerated vocal prosody, is hypothesized to pro
vide cues of safety to the client. Hypothetically, these cues are processed, via neuroception, and reflexively recruit and modulate the neural regulation of the middle- ear muscles. Based on the theory, this process would functionally reduce auditory hypersensitivities, stimulate spontaneous social engagement, and calm physiological state by increasing the influence of ventral vagal pathways on the heart. The intervention stimuli are listened to on headphones. The protocol consists of 60 minutes of listening on five consecutive days in a quiet room without major distractors, while the clinician, parent, or researcher provides social support to ensure that the participant remains calm. The neurophysiological basis of the intervention is elaborated in other publications (see Porges, 2011; Porges & Lewis, 2010).
Since the late 1990s my research group has been evaluating and refining the protocol. We have tested the protocol on several hundred children with a variety of disorders including children with autism spectrum disorders, speech/language delays, auditory hypersensitivities, and behavioral regulation disorders. The outcomes have been positive with noticeable increases in spontaneous social engagement behaviors, reduced sound sensitivities, improved organization of social behaviors and emotional state, and improved and more spontaneous verbal communication highlighted by more expressive voices. We have also conducted and published two peer- reviewed publications describing our findings (see Porges et al., 2013; Porges et al., 2014). During the past few years we have organized several clinical trials, which are currently registered on ClinicalTrials.gov. These new clinical trials are evaluating the intervention with different populations including children with abuse histories, individuals with attention and concentration difficulties, and children with Prader Willi Syndrome.
Since the release of the Safe and Sound Protocol, we have received feedback from therapists that matches the positive behavioral changes in children that we observed in our research. During the 20 years that we have tested the Safe and Sound Protocol with children, we have not observed any major adverse effects. Occasionally, we have observed an initial tactile sensitivity to the headphones, which would rapidly resolve. Also, perhaps due to previous unpleasant experiences with sounds and headphones, the combination of sounds and context might provoke minor anxiety in the child, which has rapidly resolved. This success is, in part, due to the “safe” context in which the intervention is delivered. For children, the safe context is efficiently structured by creating a ‘safe’ clinical environment with a therapist who projects welcoming cues of warmth to the child. This sense of a ‘safe container’ is supported by a safe and protective parent or caregiver accompanying the child, while the child experiences the Safe and Sound Protocol.
The Safe and Sound Protocol as an intervention has two components: first, structuring a safe context in which the intervention is delivered; and second, delivering the acoustic features of the sound presented during the intervention that serve as a neural exercise. The safe component is managed by the practitioner delivering the intervention. The sound component is embedded in the acoustic stimuli. It is important to acknowledge successful implementation of the intervention requires both components. For the Safe and Sound Protocol to be effective, it is necessary to maintain the client’s nervous system in a state of safety.
For the Safe and Sound Protocol to be effective with adults, similar to applications with children, it is necessary to maintain the nervous system in a state of safety. Maintaining the adult’s nervous system in a state of safety may be challenging, especially adults with trauma histories. Unlike the ‘safe containment’ that children experience in the presence of a caring and supportive adult, adults frequently arrive at a clinic without a supportive partner. Suggesting that a trusted friend, who would be available for support and regulation, accompany the client would be helpful in maintaining the client in a state of safety. Vulnerability to state changes might be exacerbated if the adult comes alone to the clinic.
Emotional and physiological reactions to the intervention are a potent signal that the stimuli are effectively triggering neural circuits. However, for the stimuli to trigger and exercise neural circuits that promote spontaneous social communication, improved state regulation, and reduced auditory hypersensitivities, the nervous system has to be in a safe state. More accurately, the nervous system has to feel protected and sufficiently trusting not to move into states of self- protection, hypervigilance, and defense. This may require a titration of the acoustic stimuli with the client temporarily pausing the intervention stimuli when the sounds elicit a strong emotional or visceral reaction. If the client feels discomfort, the client should be empowered to pause the intervention to allow their nervous system to stabilize. Although the fixed protocol works extremely well with children, adults may have a complicated history and may have difficulties feeling safe. As we move into the treatment of adults, we will continue to learn through the detailed comments from the therapists about variations in responses. This important feedback will allow us to modify the protocol to optimize the client’s outcome
Passive and Active Pathways
The human nervous system provides two pathways to trigger neural mechanisms capable of downregulating defense and enabling states of calmness that
support health, spontaneous social behavior, and connectedness. One pathway is passive and does not require conscious awareness (see neuroception), and the other is active and requires conscious voluntary behaviors to trigger specific neural mechanisms that change physiological state (see neural exercise).
Both the passive and active pathways regulate the social engagement system. The passive pathway recruits the social engagement system through cues of safety such as a quiet environment, positive and compassionate therapist- patient interactions, prosodic quality (e.g., melodic intonation) of the therapist’s vocalizations, and music modulated across frequency bands that overlap with vocal signals of safety used by a mother to calm her infant. Successful therapists, regardless of their orientation, often intuitively manipulate the passive pathway in treatment. In contrast, the active pathway recruits the social engagement system when the patient engages in reciprocal dialogue and other practices, such as vocalizations, voluntarily controlled breathing, movements, or postures. Access to the client’s active pathway is dependent on the passive pathway effectively triggering a state of safety in the client.
The passive pathway is an effective and efficient method to recruit the social engagement system to spontaneously transition the client into a ventral vagal state. The passive pathway provides the client with feelings of safety. The active pathway provides the neural exercises to empower the client to efficiently move into and out of a ventral vagal state. Through effective interventions the client may have transitory experiences in states previously associated with defense and dominated by either the sympathetic nervous system or the dorsal vagus. The exercises enable the client to functionally contain previously disruptive autonomic states by accessing the social engagement system and the ventral vagus. The passive pathway provides the client with feelings of safety, while the active pathway challenges these feelings of safety by ‘exercising’ the neural resources of the social engagement system. These sequential processes expand resilience and provide resources to calm, to co- regulate, and self- regulate when challenged.
Polyvagal Theory: Trauma Only Makes Sense in the Light of Evolution
At the core of the evolved features that define mammals is the role that social interaction plays in their survival. Functionally, the ability to establish feelings of safety within a social interaction underlies their survival and acts as a prepotent biological imperative. This important attribute and a refinement of the meaning of “survival of the fittest” was emphasized by the evolutionary biologist Dobzhansky (1962), when he stated that “the fittest may also be the
gentlest, because survival often requires mutual help and cooperation.” For the survivors of trauma, their lives reflect a loss of these mammalian qualities.
As Polyvagal Theory has deconstructed several of the mechanisms through which trauma retunes the nervous system, an understanding of evolution and dissolution provides insights into the physiological and psychological experiences and helps the client generate a plausible personal explanatory narrative. This emphasis on evolution in understanding trauma reactions is reminiscent of Dobzhansky’s most famous quote, “Nothing in biology makes sense except in the light of evolution” (1973, p. 125). Consistent with Dobzhansky, a polyvagal perspective explicitly assumes that the response to trauma only make sense in the light of evolution.
Synthesis
Polyvagal Theory emphasizes that humans, similar to other mammals, consist of a collection of dynamic, adaptive, interactive, and interdependent physiological systems. From this perspective, it becomes apparent that the autonomic nervous system cannot be treated as functionally distinct from the central nervous system. Consistent with Polyvagal Theory, the heart and other organs are not “floating in a visceral sea,” but are metaphorically anchored to central structures by motor pathways and continuously signaling central regulatory structures via an abundance of sensory pathways. This dynamic, bidirectional communication between brain structures and bodily organs influences mental state, biases perception of the environment, prepares the individual to be either welcoming or defensive of others. These processes simultaneously support or disrupt health, growth, and restoration.
The theory provides a plausible explanation of how a response to life threat could retune the autonomic nervous system to lose resilience and to remain in defense states. This retuning might lead to disruptions in homeostatic function with manifestations in visceral organs (e.g., heart disease, irritable bowel) or diffuse symptoms of dysregulation (e.g., fibromyalgia, dysautonomia), while simultaneously limiting access to the social engagement system that would compromise the ability to co- regulate through social interactions. These common consequences of trauma are highlighted by difficulties in feeling connected and safe with others. Polyvagal Theory explains how both aspects of disruption (i.e., lack of safety with others and disorders of bodily organs) are manifestations of a retuned autonomic nervous system, and offers insights into rehabilitation. Thus, Polyvagal Theory provides an optimistic strategy for therapy, which would be based on a “retuning” of the autonomic nervous system through portals of the social engagement system.
References
Ben- Menachem, E. (2001). Vagus nerve stimulation, side effects, and long- term safety. Journal of Clinical Neurophysiology, 18(5), 415– 418.
Carter, C. S. (1998). Neuroendocrine perspectives on social attachment and love. Psychoneuroendocrinology, 23(8), 779– 818.
Clauw, D. J. (2014). Fibromyalgia: A clinical review. JAMA, 311(15), 1547– 1555.
Craig, A. D. (2005). Forebrain emotional asymmetry: A neuroanatomical basis? Trends in Cognitive Sciences, 9(12), 566– 571.
Dobzhansky, T. G. (1962). Mankind evolving: The evolution of the human species. The Eugenics Review, 54(3), 168– 169.
Dobzhansky, T. G. (1973). Nothing in biology makes sense except in the light of evolution. The American Biology Teacher, 35(3), 125– 129.
Groves, D. A., & Brown, V. J. (2005). Vagal nerve stimulation: A review of its applications and potential mechanisms that mediate its clinical effects. Neuroscience & Biobehavioral Reviews, 29(3), 493– 500.
Hess, W. (1949/2014). The central control of the activity of internal organs. Nobelprize.org. Retrieved from http://www.nobelprize.org/nobel_prizes/medicine/laureates/1949/hess- lecture.html
Howland, R. H. (2014). Vagus nerve stimulation. Current Behavioral Neuroscience Reports, 1(2), 64– 73.
Jackson, J. H. (1882). On some implications of dissolution of the nervous system. Med Press Circ, 2, 411– 414.
Kolacz, J. K., Lewis, G. F., & Porges, S. W. (In press). The integration of vocal communication and biobehavioral state regulation in mammals: A polyvagal hypothesis. In S. M. Brudzynski (Ed.), Handbook of ultrasonic vocalization. London, England: Elsevier.
Mazurak, N., Seredyuk, N., Sauer, H., Teufel, M., & Enck, P. (2012). Heart rate variability in the irritable bowel syndrome: A review of the literature. Neurogastroenterology and Motility, 24(3), 206– 216.
Ogden, P., Minton, K., & Pain, C. (2006). Trauma and the body: A sensorimotor approach to psychotherapy. New York, NY: Norton.
Porges, S. W. (1995). Orienting in a defensive world: Mammalian modifications of our evolutionary heritage. A Polyvagal Theory. Psychophysiology, 32(4), 301– 318.
Porges, S. W. (2001). The Polyvagal Theory: Phylogenetic substrates of a social nervous system. International Journal of Psychophysiology, 42(2), 123– 146.
Porges, S. W. (2003). Social engagement and attachment. Annals of the New York Academy of Sciences, 1008(1), 31– 47.
Porges, S. W. (2004). Neuroception: A subconscious system for detecting threats and safety. Zero to Three (J), 24(5), 19– 24.
Porges, S. W. (2007). The polyvagal perspective. Biological Psychology, 74(2), 116– 143.
Porges, S. W. (2009). The Polyvagal Theory: New insights into adaptive reactions of the autonomic nervous system [Supplementary material]. Cleveland Clinic Journal of Medicine, 76, S86.
Porges, S. W. (2011). Norton Series on Interpersonal Neurobiology. The Polyvagal Theory: Neurophysiological foundations of emotions, attachment, communication, and self- regulation. New York, NY: Norton.
Porges, S. W., Bazhenova, O. V., Bal, E., Carlson, N., Sorokin, Y., Heilman, K. J., Cook, E. H., & Lewis, G. F. (2014). Reducing auditory hypersensitivities in autistic spectrum dis
order: Preliminary findings evaluating the listening project protocol. Frontiers in Pediatrics. doi:10.3389/fped.2014.00080
Porges, S. W., & Lewis, G. F. (2010). The polyvagal hypothesis: Common mechanisms mediating autonomic regulation, vocalizations and listening. Handbook of Behavioral Neuroscience, 19, 255– 264.
Porges, S. W., Macellaio, M., Stanfill, S. D., McCue, K., Lewis, G. F., Harden, E. R., . . . Heilman, K. J. (2013). Respiratory sinus arrhythmia and auditory processing in autism: Modifiable deficits of an integrated social engagement system? International Journal of Psychophysiology, 88(3), 261– 270.
Porges, S. W., Bazhenova, O. V., Bal, E., Carlson, N., Sorokin, Y., Heilman, K. J., Cook, E. H., & Lewis, G. F. (2014). Reducing auditory hypersensitivities in autistic spectrum disorder: preliminary findings evaluating the listening project protocol. Frontiers in Pediatrics. doi:10.3389/fped.2014.00080
Reed, S. F., Ohel, G., David, R., & Porges, S. W. (1999). A neural explanation of fetal heart rate patterns: A test of the Polyvagal Theory. Developmental Psychobiology, 35(2), 108– 118.
Sanossian, N., & Haut, S. (2002). Chronic diarrhea associated with vagal nerve stimulation. Neurology, 58(2), 330– 330.
Siegel, D. J. (1999). The developing mind (Vol. 296). New York, NY: Guilford Press.
Staud, R. (2008). Heart rate variability as a biomarker of fibromyalgia syndrome. Future Rheumatology, 3(5), 475– 483.
Thayer, J. F., & Lane, R. D. (2000). A model of neurovisceral integration in emotion regulation and dysregulation. Journal of Affective Disorders, 61(3), 201– 216.
Thayer, J. F., & Lane, R. D. (2007). The role of vagal function in the risk for cardiovascular disease and mortality. Biological Psychology, 74(2), 224– 242.
Whitehead, W. E., Palsson, O. S., Levy, R. R., Feld, A. D., Turner, M., & Von Korff, M. (2007). Comorbidity in irritable bowel syndrome. The American Journal of Gastroenterology, 102(12), 2767– 2776.
Stephen W.Porges